|
|
Line 442: |
Line 442: |
| <font color="#003366"><a href="http://bcs.useoul.edu/b/JC/bliss%20lomo.pdf" alt="bless lomo"></a>Long-lasting potentiation of synaptic transmission in the dentate area of the anaesthetized rabbit following stimulation of hte perforant path </a></font></h4> | | <font color="#003366"><a href="http://bcs.useoul.edu/b/JC/bliss%20lomo.pdf" alt="bless lomo"></a>Long-lasting potentiation of synaptic transmission in the dentate area of the anaesthetized rabbit following stimulation of hte perforant path </a></font></h4> |
| | | |
- | 1.The after-effects of repetitive stimulation of the perforant path fibres to the dentate area of the hippocampal formation have been examined with extracellular micro-electrodes in rabbits anaesthetized with urethane.<br>
| + | - The after-effects of repetitive stimulation of the perforant path fibres to the dentate area of the hippocampal formation have been examined with extracellular micro-electrodes in rabbits anaesthetized with urethane.<br> |
- | 2.In fifteen out of eighteen rabbits the population response recordedfrom granule cells in the dentate area to single perforant path volleys was potentiated for periods ranging from 30 min to 10 hr after one or more conditioning trains at 10-20/sec for 10-15 sec, or 100/sec for 3-4 sec.<br>
| + | - In fifteen out of eighteen rabbits the population response recordedfrom granule cells in the dentate area to single perforant path volleys was potentiated for periods ranging from 30 min to 10 hr after one or more conditioning trains at 10-20/sec for 10-15 sec, or 100/sec for 3-4 sec.<br> |
- | 3.The population response was analysed in terms of three parameters:
| + | - The population response was analysed in terms of three parameters: |
| the amplitude of the population excitatory post-synaptic potential (e.p.s.p.), signalling the depolarization of the granule cells, and the amplitude and latency of the population spike, signalling the discharge of the granule cells.<br> | | the amplitude of the population excitatory post-synaptic potential (e.p.s.p.), signalling the depolarization of the granule cells, and the amplitude and latency of the population spike, signalling the discharge of the granule cells.<br> |
- | 4.All three parameters were potentiated in 29% of the experiments;
| + | - All three parameters were potentiated in 29% of the experiments; |
| in other experiments in which long term changes occurred, potentiation was confined to one or two of the three parameters. A reduction in the latency of the population spike was the commonest sign of potentiation, occurring in 57 % of all experiments. The amplitude of the population e.p.s.p. was increased in 43 %, and of the population spike in 40 %, of all experiments.<br> | | in other experiments in which long term changes occurred, potentiation was confined to one or two of the three parameters. A reduction in the latency of the population spike was the commonest sign of potentiation, occurring in 57 % of all experiments. The amplitude of the population e.p.s.p. was increased in 43 %, and of the population spike in 40 %, of all experiments.<br> |
- | 5.During conditioning at 10-20/sec there was massive potentiation of the population spike ('frequency potentiation'). The spike was suppressed during stimulation at 100/sec. Both frequencies produced long-term potentiation.<br>
| + | - During conditioning at 10-20/sec there was massive potentiation of the population spike ('frequency potentiation'). The spike was suppressed during stimulation at 100/sec. Both frequencies produced long-term potentiation.<br> |
- | 6.The results suggest that two independent mechanisms are responsiblefor long-lasting potentiation: (a) an increase in the efficiency of synaptic transmission at the perforant path synapses; (b) an increase in the excitability of the granule cell population.
| + | - The results suggest that two independent mechanisms are responsiblefor long-lasting potentiation: (a) an increase in the efficiency of synaptic transmission at the perforant path synapses; (b) an increase in the excitability of the granule cell population. |
| </html> | | </html> |
| | | |
Revision as of 07:19, 10 June 2010
Goal
- To understand how the mind, behavior and brain are interrelated by linking key cognitive & emotional functions to neural activities at multiple levels of brain systems.
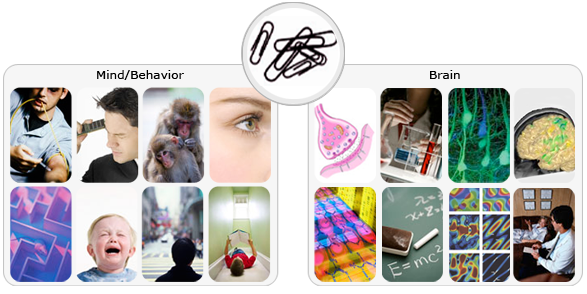
Plans
The Strategy of Linking Brain and Mind (SLBM)
First, we have identified a set of comprehensive but focused set of cognitive functions and targeted
brain mechanisms with an aim to achieve breadth while insuring that our studies fall squarely within the
areas of expertise of our associated faculty (Fig 1A, 1B). Second, to address the 'linking' questions
around those key cognitive and brain mechanisms, we intend to integrate cutting-edge ‘neurometric'
techniques to probe neural activities/structures at multiple levels spanning the depth of genetic, molecular,
synaptic, neuronal, local/global network-level measurements (Fig 1C). These brain measurements will be
paralleled by state-of-the-art 'psychometric' tools to capture mind in action, with behavioral tasks
spanning the breadth of behavioral genetics, rodent memory tasks, visual perception tasks, eye movements,
reverse correlation and social perception tasks (Fig 1D). Third, based on the neurometric and
psychometric data, we will build tight linkages between brain activity/structure and mind. In doing so,
computational approaches will help us to build comprehensive neural models constrained by data from
empirical studies (Fig 1E). Computational models of complex brain systems allow us to put to test, via
simulation, predictions that cannot be tested in empirical situations and to guide empirical studies by
generating testable predictions about behaviors in the domains of both brain and mind. In addition, we
will study brains and minds in abnormal states by specifying the roles of neural circuits underlying
cognitive deficits in patients with psychiatric disorders and in individuals with remarkable cognitive
abilities that fall outside the range of normal (Fig 1F). Our multi-level approach, transpiring through
'gene', 'protein', 'neuron', 'system', 'behavior', 'abnormality' and 'computation', can lead to
fundamental cure for mental diseases in the future.
step 1 Identification of key Cognitive & Brain mechanisms
Perirhinal cortex / Entorhinal cortex / Parietal cortex /
Hippocampus / Amygdala / Visual cortex / Prefrontal
cortex / Corpus callosum / Orbitofrontal cortex / Longrange
cortical circuitry
|
|
step 2 Integration of key Neurometric & Psychometric tools

step 3 Linking Neural Activities to Cognitive Functions
Research Units
Three Core Research Units
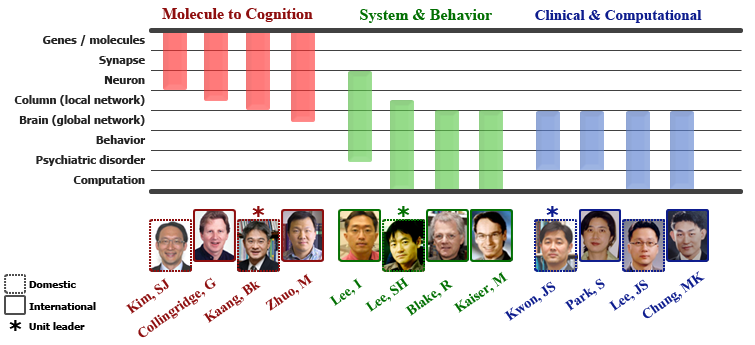
Research overview
I. Introduction and overview of research plans
Our research aim is to use biological, behavioral and computational methods to understand how the
mind, brain and behavior are interrelated. Studying humans and animals, our research will link key
cognitive and emotional functions to neural activities at multiple levels of brain systems. To establish
these linkages, we set forth a research strategy, named 'SLBM (Strategy of Linking Brain and Mind)', the
major steps and components of which are summarized in the following three sections.
Target mechanisms |
|
|
Synaptic plasticity |
 |
|
|
Calcium sensors |
 |
|
|
Perirhinal cortex |
 |
 |
 |
Entorhinal cortex |
 |
 |
 |
Auditory cortex |
 |
 |
|
Somatosensory cortex
Parietal cortex |
|
 |
|
Prefrontal cortex |
 |
 |
 |
Hippocampus |
 |
 |
 |
Amygdala |
|
 |
 |
Visual cortex |
|
 |
 |
Anterior cingulatu cortex |
|
 |
 |
Basal ganglia |
|
 |
 |
Superior temporal cortex |
|
 |
 |
Long-range cortical circuitry |
|
|
 |
Orbitofrontal cortex |
|
|
 |
Ventromedial frontal cortex |
|
|
 |
Corpus callosum |
|
|
 |
|
Episodic Memory |
 |
 |
 |
Contextual Memory |
 |
 |
 |
Place Memory |
 |
 |
 |
Association Memory |
 |
 |
 |
Working Memory |
 |
 |
 |
Emotion |
 |
|
 |
Pain and Pleasure |
 |
|
|
Attention |
 |
 |
 |
Anxiety |
 |
 |
 |
Motion and form perception |
|
 |
 |
Perceptual organization |
|
 |
 |
Decision making |
 |
 |
 |
Multistable perception |
|
 |
 |
Adaptation |
|
 |
|
Cross-modal sensory integration |
|
 |
|
Social interaction |
|
|
 |
|
Measurements |
|
|
Transgenic animals |
 |
|
|
Protein-protein interaction |
 |
|
|
Patch-clamp recording |
 |
|
|
In vitro electrophysiology |
 |
|
|
in vivo electrophysiology |
 |
 |
|
Two-photon imaging |
 |
 |
|
Multi-unit parallel recording |
|
 |
|
Chronic eletrophysiology |
|
 |
|
Lesion/inactivation study |
|
 |
|
(F)MRI/DTI/PET/MEG |
|
 |
 |
Classification of cortical activity |
|
 |
 |
Functional connectivity analysis |
|
 |
 |
Transcranial magnetic stim |
|
 |
 |
Brain Segmentation |
|
 |
 |
Near-Infrared Optical Imaging |
|
|
 |
Tractography |
|
|
 |
|
Maze learning tasks |
 |
 |
|
Fear conditioning |
 |
 |
|
Anxiety and depression test |
 |
 |
|
Test of genetic mutant mice |
 |
 |
|
Behavioral genetics |
 |
 |
|
Bistable perception task |
|
 |
|
Signal detection task |
|
 |
|
2-alternative force choice task |
|
 |
|
Motion discrimination task |
|
 |
|
Reverse correlation |
|
 |
|
Biological motion task |
|
 |
 |
Visual search task |
|
 |
 |
Eye movements |
|
 |
 |
Face recognition task |
|
 |
 |
Neuropsychological testing |
|
 |
 |
Theory-of-Mind task |
|
|
 |
Social cue Perception task |
|
|
 |
Imitation task |
|
|
 |
|
|
|
|
|
Neural modeling of dynamic vs resting state network/
Hippocampal network of memory/Propagation of cortical excitability/Motion perception/Oscillations
in brain or percept/Cortical hippocampal interaction
|
|
Neurocognitive deficits in Schizophrenia/Depression/Amnesia/Autism/
Alzheimer's Disease/Epilepsy/Synesthesia/Phantom pain/ Anxiety/Fragile X disease
|
|
Protein to Cognition unit System & Cognitive unit Clinical & Computational unit
Figure 1. Structure of SLBM |
|
Publication
WCU Publication
Long-lasting potentiation of synaptic transmission in the dentate area of the anaesthetized rabbit following stimulation of hte perforant path
- The after-effects of repetitive stimulation of the perforant path fibres to the dentate area of the hippocampal formation have been examined with extracellular micro-electrodes in rabbits anaesthetized with urethane.
- In fifteen out of eighteen rabbits the population response recordedfrom granule cells in the dentate area to single perforant path volleys was potentiated for periods ranging from 30 min to 10 hr after one or more conditioning trains at 10-20/sec for 10-15 sec, or 100/sec for 3-4 sec.
- The population response was analysed in terms of three parameters:
the amplitude of the population excitatory post-synaptic potential (e.p.s.p.), signalling the depolarization of the granule cells, and the amplitude and latency of the population spike, signalling the discharge of the granule cells.
- All three parameters were potentiated in 29% of the experiments;
in other experiments in which long term changes occurred, potentiation was confined to one or two of the three parameters. A reduction in the latency of the population spike was the commonest sign of potentiation, occurring in 57 % of all experiments. The amplitude of the population e.p.s.p. was increased in 43 %, and of the population spike in 40 %, of all experiments.
- During conditioning at 10-20/sec there was massive potentiation of the population spike ('frequency potentiation'). The spike was suppressed during stimulation at 100/sec. Both frequencies produced long-term potentiation.
- The results suggest that two independent mechanisms are responsiblefor long-lasting potentiation: (a) an increase in the efficiency of synaptic transmission at the perforant path synapses; (b) an increase in the excitability of the granule cell population.
An Animal Model of a Behavioral Intervention for Depression
Although conditioned inhibition of fear (or learned safety) is a learning process critical for preventing chronic stress, a predisposing factor for depression and other psychopathologies, tittle is known about its functional purposes or molecular mechanisms. To obtain better insight into learned safety, we investigated its behavioral and molecular characteristics and found that it acts as a behavioral antidepressant in two animal models. Learned safety promotes the survival of newborn cells in the dentate gyrus of the hippocampus, while its antidepressant effect is abolished in mice with ablated hippocampal neurogenesis. Learned safety also increases the expression of BDNF in the hippocampus and leads to downregulation of genes involved in the dopaminergic and neuropeptidergic but not the serotonergic system in the basolateral amygdala. These data suggest that learned safety is an animal model of a behavioral antidepressant that shares some neuronal hallmarks of pharmacological antidepressants but is mediated by different molecular pathways.
Distinct Neural Bases of Route Following and Wayfinding in Humans An Animal Model of a Behavioral Intervention for Depression
Finding one's way in a large-scale environment may engage different cognitive processes than following a familiar route. The neural bases of these processes were investigated using functional MRI (fMRI). Subjects found their way in one virtual-reality town and followed a well-learned route in another. In a control condition, subjects followed a visible trail.Within subjects, accurate wayfinding activated the right posterior hippocampus. Between-subjects correlations with performance showed that good navigators (i.e., accurate wayfinders) activated the anterior hippocampus during wayfinding and head of caudate during route following. These results coincidewith neurophysiological evidence for distinct response (caudate) and place (hippocampal) representations supporting navigation. We argue that the type of representation used influences both performance and concomitant fMRI activation patterns.